Optimise automotive sensing with Hall effect sensors
Improving the safety of vehicle drivers and passengers is receiving ever greater emphasis, with automotive engineers deploying sensing devices in their systems that deliver increased sophistication and set higher performance benchmarks.
By Dieter Verstreken, Melexis.
A multitude of different design and integration pressures must be considered, meaning for such sensors to be effective they must not impact heavily on the engineering overhead, the financial investment involved, or bring weight penalties that impact efficiencies. This article will describe some of the demands being placed on automobile design projects and show how dynamics now in place call for specification of a new type of sensor.
Mechanical components, such as magnetic reed switches and precious metal contact switches, were widely used in certain automotive sensing applications for several decades. While relatively cheap and simple to incorporate, with new opportunities for sensor technology in automobiles emerging, they are reaching technical limitations. Automotive safety standards now require, for example, knowledge of seat position and whether the belt buckle mechanism is latched or not. Though reed switches are in principle capable of executing such tasks, increasingly mechanical devices of this kind are found to be lacking. This manifests itself in several key areas, such as the depth of functionality offered, compactness and assembly process robustness. The typical working environments for these devices expose the switches to build-up of dirt, grease, pet hair and food/drink spillages. Mechanical switches, which are reliant on moving parts, are more prone to failure in such settings and have shorter lifespans as a result. Therefore their potential is somewhat limited as the scope for sensing systems in vehicles continues to rapidly grow. For example seat buckle latch detection mechanisms and load cells can be utilised to check vehicle occupancy for airbag deployment purposes. Conventional mechanical switches are not reliable enough to be fully effective in this sort of application.
A non-contact, solid state solution is, for a number of reasons, far more attractive - offering an extremely streamlined way of capturing required position data with boosted ruggedness and diagnostic messaging of proper function. Though optoelectronic devices present one non-contact alternative to mechanical implementation, their effectiveness can still be marred by exposure to contaminants. Hall effect sensors, however, thanks to the greater accuracy and responsiveness they exhibit, plus their robust construction, have the attributes needed to supplant reed switches in many automotive position sensing applications.
Figure 1 - schematic of seat positioning sensor system
Magnetic sensors can be in used seat positioning, for instance, to ascertain if the front seat is close to the dashboard, in a collision this critical information directs the airbag controller to inflate the airbag with less force than if the seat were placed further back. Figure 1 describes a typical seat position sensor system, based on a magnetic sensing mechanism. The ferrous metal frame of the seat trigger’s the magnetic sensor, which means that the engineer has to rely on a back-biased approach, with the sensor and magnet mounted into a single module and the module then mounted onto the seat track. Movement along the track alters the magnetic field lines and this is picked up by the sensor.
For any form of magnetic sensor within automotive environments it is necessary to set acceptable tolerances, as there is a lot of variation in operating conditions to factor in. This is difficult however and can compromise the effectiveness of the sensor’s operation. Each sensor being used for a different function in the automotive system will require the setting of switch thresholds that are relevant to the particular application. Rather than stocking an extensive inventory of different Hall effect sensors, all of which have very similar functional attributes, a costly and logistically challenging exercise, it would be better to have sensor platform that can be custom programmed for different uses. There is as a result a clear need for a more versatile approach which has provision for supporting numerous different magnetic actuations.
The advent of programmable sensors, based on automotive grade non-volatile memory technology has already proved to be of great benefit to the automotive industry. Through these devices, support for in-situ programming can be offered to engineering teams. Prototyping greatly simplifies the engineering phase while production benefits from the improved repeatability, compensation for mechanical assembly tolerance variation and reduced inventory part types.
The sensing systems for which the sensors are constituent parts can thereby be optimised for particular functional requirements. This enables migration to cheaper ferrite magnetic materials as well as providing engineers with the ability to compensate for thermal drift (as certain magnets become weaker when the ambient temperature rises). While a mechanical switch can only distinguish between a current being present or no current at all, a programmable magnetic sensor has the advantage of supporting full diagnostics. It can determine a variety of different current states. There is, as a result, better process in place not just for identifying that a problem has arisen, but also determining the exact nature of that problem (e.g. short circuits, broken wires causing open circuits, etc.).
As we have seen for back biasing applications, such as seat positioning sensors, a degree of programmability is needed in order to define the range and resolution of the magnetic thresholds, as well as thermal sensitivity coefficients. Implementation of programmable Hall sensors enables this to be accomplished, but unfortunately it can increase system complexity and raise the overall bill of materials costs. Employing sensor devices with 2-wire rather than 3-wire interfaces can bring about marked reductions in the cabling and the component count. This decreases the associated cost, shortens implementation time and utilises less space. 2-wire magnetic sensor ICs allow the reuse of the cable wire harness that was previously designed for the incumbent mechanical switch device, thereby easing upgrades - with improved performance and greater reliability but no change to the basic set up.
Figure 2 - comparison between 3-wire & 2-wire Hall sensor implementations
Fabricated on a proprietary submicron mixed signal CMOS technology, Melexis‘ MLX92242 Hall latch/switch, unlike other Hall sensor devices, supports 2-wire implementation. It includes a voltage regulator, Hall sensing element, non-volatile memory, diagnostic circuitry and a current sink-configured output driver all in a single package. Via the built-in EEPROM memory it is possible to carry out end-of-line calibration, so that during the vehicle production process all the various tolerances that will affect the sensor’s operation in relation to a particular application can be dealt with. Once programmed the memory can be locked. The temperature compensation afforded by this device counterbalances the influence of magnetic drift. This means it can be used with all kinds of magnets (including the low cost ferrite materials) with the expense associated with position, proximity detection and encoding applications thus being curbed.
The MLX92242 still provides all the necessary diagnostic capacity to respond accordingly a malfunction condition arise, but only requires use of the VDD and GND pins. This means that it can facilitate modernisation of a sensor system - so that bulky, less reliable mechanical switches can be dispensed with while not calling for any alteration in the existing infrastructure. Alternatively when implemented into brand new automotive designs it can help vehicle manufacturer to deploy sensing systems requiring far less cabling - with space, weight and cost advantages. The offset cancellation system within the magnetic core of the MLX92242 enables faster and more precise processing of the data acquired from the sensing element while being resilient to temperature fluctuations and other forms of stress. On-chip thermal protection disables the output if the junction temperature increases above a pre-set value and automatically recovers once the temperature decreases below a safe value.
Figure 3 - functional block diagram from the Melexis MLX92242
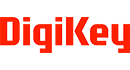