Redesigning RTD-based temperature sensors for the smart factory age
The old saying 'if it ain’t broke, don’t fix it' cautions against tampering with something that performs reliably and regularly proves its worth. Arguably, this advice applies to the circuit design used in many RTD sensors that quietly and efficiently measure temperature in industrial manufacturing facilities worldwide.
This article originally appeared in the Oct'23 magazine issue of Electronic Specifier Design – see ES's Magazine Archives for more featured publications.
However, to meet the requirements of Industry 4.0, factories are becoming smarter, and it is becoming clear that many current RTD sensors will not be fit for purpose in these environments. Smaller form factors, flexible communications, and remote configurability are some features that automation engineers now demand from industrial temperature sensors, but incumbent solutions cannot support them. This article revisits the building blocks used in the design of many RTD-based temperature sensors and discusses the limitations that these impose on a sensor’s application. It then shows how to quickly redesign this type of sensor to equip it with the features required in this new industrial age.
Figure 1. An example of an RTD-based temperature sensor
Temperature sensor building blocks
The building blocks of an RTD industrial temperature sensor are shown in Figure 2.
Figure 2. A block diagram of an RTD industrial temperature sensor
An RTD translates a physical quantity (temperature) into an electrical signal and is typically used to detect temperatures between –200 and +850°, having a highly linear response over this temperature range. Metal elements commonly used in RTDs include nickel (Ni), copper (Cu), and platinum (Pt), with Pt100 and Pt1000 platinum RTDs being the most common. An RTD can consist of either two, three, or four wires, but the 3-wire and 4-wire versions are the most popular. Since they are passive devices, RTDs require an excitation current to produce an output voltage. This can be generated using a voltage reference, buffered by an operational amplifier that drives current into the RTD to produce an output voltage signal that varies in response to changes in temperature. This signal varies from tens to hundreds of millivolts depending on the type of RTD used and the measured temperature, as shown in Figure 3.
The AFE amplifies and conditions the low amplitude RTD signal before the analog-to-digital converter (ADC) digitises it for the microcontroller to run an algorithm to compensate for any nonlinearity it contains. This sends the digital output to a process controller via a communications interface. The AFE is commonly implemented using a signal chain of components in which each performs a dedicated function, as shown in Figure 4.
Many existing temperature sensor designs use this discrete approach that requires a printed circuit board (PCB) large enough to accommodate the footprint of all the integrated circuits (ICs) and the signal and power routing and sets a de facto minimum size for the sensor enclosure. A superior and more straightforward approach uses an integrated AFE like the AD7124-4 shown in Figure 5. This compact IC is a complete AFE in a single package and includes a multiplexer, voltage reference, programmable gain amplifier, and a sigma-delta ADC. It also provides the excitation currents for the RTD, meaning it can effectively replace five of the signal-chain components from the previous figure, significantly reducing the amount of board space required and enabling a sensor with a much smaller enclosure.
Communications interface
Most industrial sensors are designed to connect to a process controller using one (or more) industrial networks, including the many variants of field bus or Industrial Ethernet. This requires an application-specific integrated circuit (ASIC) to implement the selected network protocols. However, this approach has several disadvantages. Firstly, including a network-specific ASIC in the sensor design significantly increases cost, especially if the industrial networks are proprietary. It also limits the market for a sensor to those customers using that network. For the same sensor to work with different network protocols requires redesigning to include the necessary ASIC, which can be time-consuming, high risk, and expensive. Finally, the number and type of diagnostic features vary significantly by network type (with some providing none). Depending on the choice, it can be difficult for factory operators to identify and maintain sensors and any performance issues that arise once they have been installed in the field.
Figure 3. A voltage signal produced by a Pt100 RTD in response to increasing temperature
A better approach is to design a sensor independent of all industrial networks, thereby reducing development costs and broadening the potential customer base. This can be done using IO-Link, a 3-wire industrial communications standard that links sensors (and actuators) with all industrial control networks. In IO-Link applications, a transceiver acts as the physical layer interface to a microcontroller running the data-link layer protocol. The advantage of using IO-Link is that it carries four different types of transmissions: process data, diagnostics, configuration, and events, allowing sensors to be quickly identified, traced, and attended to if a malfunction occurs. It also enables remote configuration – for example, if the temperature threshold for a process alarm to be triggered requires changing, this can be done remotely without needing a technician to step onto the factory floor. The MAX14828 is an example of a low power, ultrasmall IO-Link device transceiver. It is available in a (4 × 4mm) 24-lead TQFN package and a (2.5 × 2.5mm) wafer-level package (WLP), allowing it to be easily integrated into an industrial RTD-based temperature (and other types of) sensor. The transceiver enables a sensor independent of the industrial network because it communicates directly with an IO-Link host installed at the process controller side, which manages communication with the interface ASIC as shown in Figure 6.
Figure 4. An AFE implemented using separate discrete components in the signal chain.
Figure 5. Implementing the AFE using the AD7124-4
Figure 6. Communication with the industrial network is performed by the IO-Link host transceiver on the controller side
Conclusion
Smart factory automation engineers have growing expectations of industrial temperature sensors, including smaller size, flexible communications, and remote configurability. This article showed how RTD temperature sensors could be quickly redesigned with a highly integrated AFE to reduce the enclosure size. It also showed how an IO-Link device transceiver allows the sensor to operate independently of the industrial network interface used to connect to a process controller. While this article focuses on RTD-based temperature sensors, this redesign can also be applied to temperature sensors that use thermistors or thermocouple transducers.
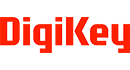